Biomechanical testing for medical device design
- thomasoverbergh
- Jan 27
- 4 min read
Updated: Feb 4
In medical device development, understanding the biomechanics of biological tissues is essential to ensure that the final product functions safely and effectively. Biomechanics involves the study of the mechanical properties of tissues like bones, tendons, ligaments, and muscles. Proper testing and analysis of these tissues, along with the materials used in medical devices, are crucial for creating devices that can withstand the stresses placed on them in the human body.
The role of biomechanics in medical device design
Biomechanics bridges engineering principles with biological systems. For medical devices, this means understanding the mechanical properties of the tissue a device is designed to replace or interact with. For example, if a device is intended to replace a bone, knowing the bone's mechanical properties is vital for predicting how the device will perform in real-world conditions. Testing biological tissues under various loading conditions allows engineers to design medical devices that are both functional and durable.
Bone structure and properties
Bone is a complex composite material made up of both solid and fluid phases. The solid phase provides structural integrity, while the fluid phase, primarily water, helps in maintaining flexibility and resilience. Bone tissues can be categorized into cortical (compact) bone and cancellous (spongy) bone, each exhibiting distinct mechanical properties.
Cortical bone is dense and forms the outer layer of bones. It has a higher Modulus of Elasticity, which helps it resist bending and twisting forces. Cancellous bone, in contrast, is more porous and less dense, providing the ability to absorb and store energy. The varying structures of cortical and cancellous bones affect their response to mechanical loads such as compression, tension, and shear.
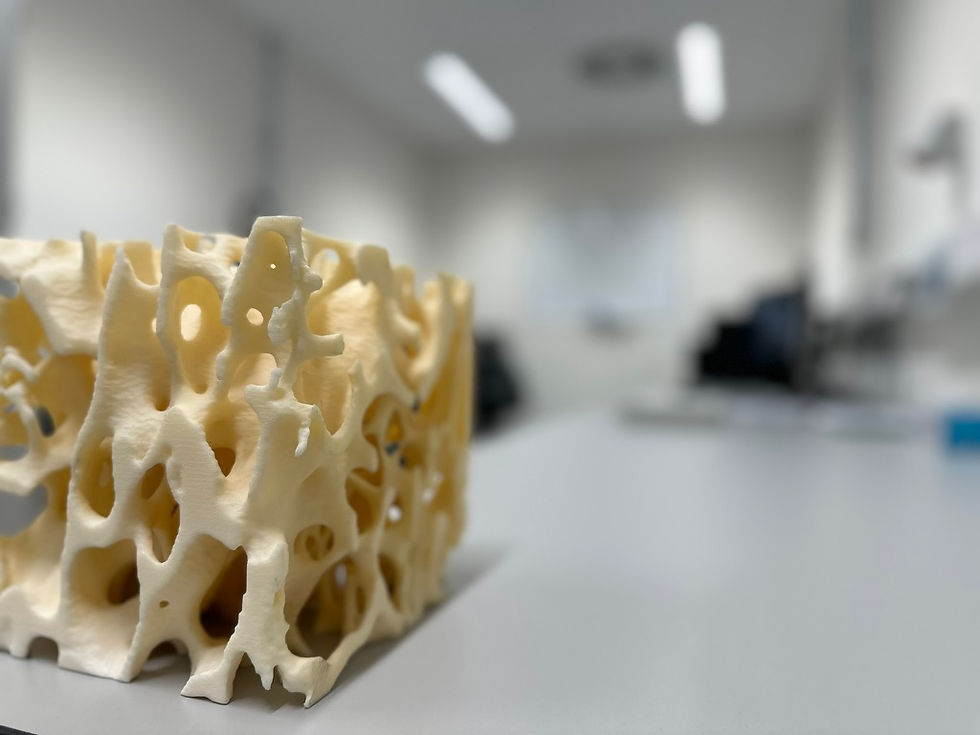
Understanding the mechanical behavior of bone
Bones exhibit different mechanical behaviors depending on factors like age, sex, and health. Additionally, the direction of applied force influences the bone's response. The stress-strain curve is often used to assess how bones react to applied forces. Initially, bone behaves elastically, meaning it returns to its original shape once the load is removed. However, once the yield point is surpassed, bones begin to undergo plastic deformation, which can lead to fracture.
Testing bone materials also involves understanding time-dependent behavior such as creep and stress relaxation. Creep refers to the slow elongation of a material under a constant load, while stress relaxation measures the decrease in stress when a material is held at a constant strain.
Key biomechanical tests for medical devices
Several tests are employed to evaluate the biomechanical properties of both biological tissues and medical devices:
Creep and recovery tests: These tests involve applying a constant load to a material for a set period and then removing it to observe how the material recovers. This is important for understanding the long-term behavior of medical implants under sustained stress.
Stress relaxation tests: Here, a material is strained to a specific point, and the stress response is measured while the strain remains constant. This test helps in understanding how materials behave when they are stretched and held in place.
Dynamic testing: This involves applying oscillatory forces to the material to measure its strain response. Dynamic tests help assess the material's ability to withstand varying forces over time, simulating real-life conditions.
In addition to these tests, the phenomenon of hysteresis — where the loading and unloading curves differ due to energy loss (often through friction) — can also be examined during dynamic mechanical testing.
Material considerations for medical devices
The choice of materials used in medical devices depends on their mechanical properties, such as Elastic Modulus, yield strength, and elongation to failure. Different materials are chosen for specific applications:
Metals and alloys: These materials are often used in load-bearing devices, such as hip and knee implants, because they have high strength and durability. However, metals may require surface treatments to prevent adverse reactions with surrounding tissues.
Ceramics: Known for their excellent compressive strength, ceramics are commonly used in dental implants. However, their poor tensile properties limit their use in applications requiring flexibility or resistance to bending forces.
Polymers: These materials offer flexibility in terms of shape and controlled degradation rates, making them suitable for a range of applications. Polymers, however, may experience wear and fatigue under repeated loading, and careful selection is needed to ensure they meet the necessary biomechanical requirements.
Standards and regulations for biomechanical testing
In the EU, medical device testing adheres to rigorous standards set by regulatory bodies to ensure safety and performance. Manufacturers must ensure that their devices meet specific biomechanical criteria based on the intended use. This includes conducting extensive mechanical testing to demonstrate that the device can withstand the forces it will encounter in the body.
Predefined ISO standards outline testing requirements for materials used in medical devices, including tests for mechanical properties, biocompatibility, and long-term durability. By following these standards, manufacturers can ensure their devices are safe, effective, and ready for clinical use.
Conclusion
Biomechanical testing is a critical part of medical device development, ensuring that implants and other devices can mimic the properties of natural tissues and withstand the stresses they will encounter in the human body. With advancements in testing methodologies and material science, designers can develop devices that provide better outcomes for patients while meeting stringent regulatory standards in the EU.
Interested how FIBEr can help? Contact us!Â